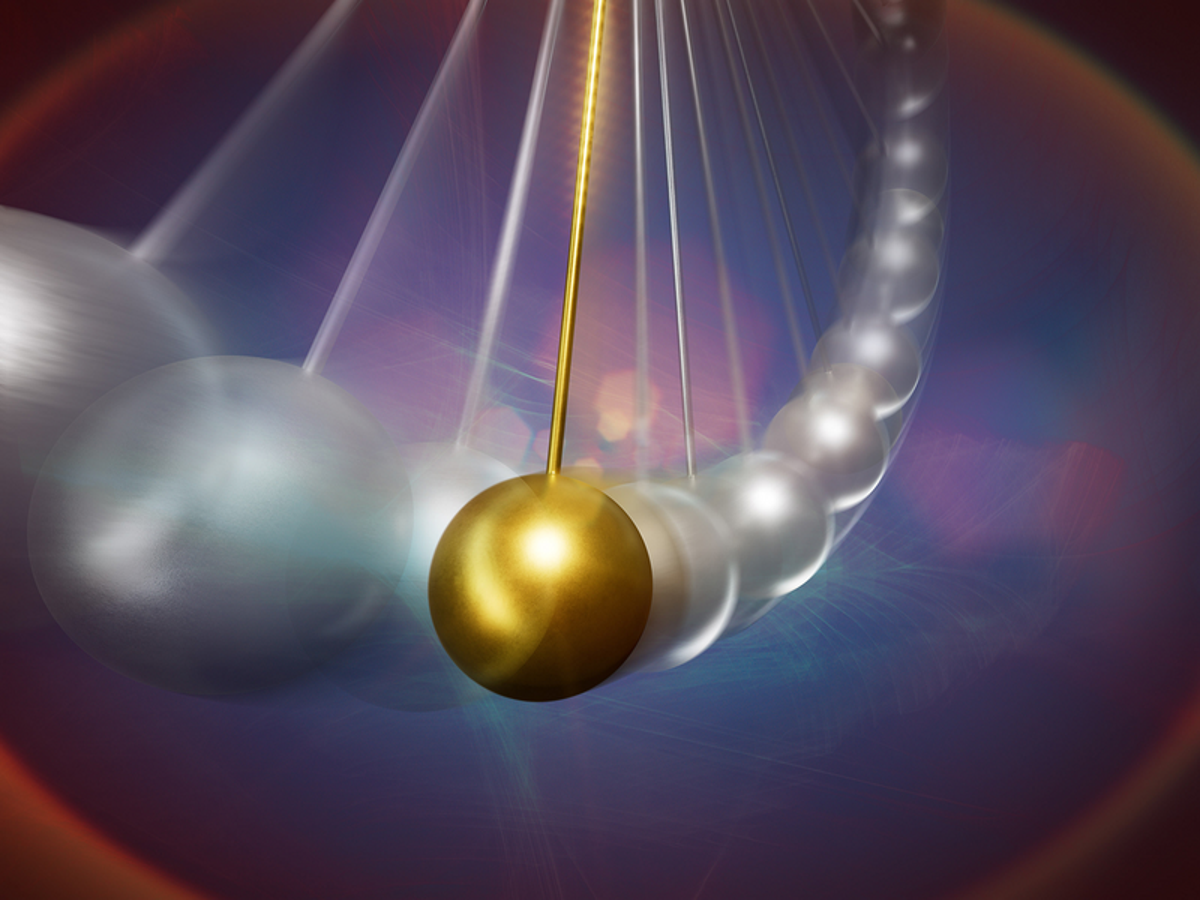
Physicists seek the dividing line between the quantum world and the classical one
There’s a rift in reality—an invisible border that separates two utterly different realms. On one side lies our everyday world, where things obey commonsense rules: objects never occupy more than one place at a time, and they exist even when we’re not looking at them. The other side is the dreamscape of quantum mechanics, where nothing is fixed, uncertainty reigns and a single atom or molecule can be in multiple places simultaneously, at least while no one is watching.
Does that mean reality has one set of laws for the macrocosm and another for the micro? Most physicists instinctively dislike the idea of a bifurcated universe. Sougato Bose, a theorist at University College London (UCL), certainly does. “My view is that quantum mechanics hasn’t been seen [at large scales] because we have not yet been able to isolate things well enough,” he says, meaning that researchers haven’t found a way to shield big objects from their environment, so their quantum properties are apparent. Like most physicists, Bose believes that quantum mechanics applies to all things great and small. He and three colleagues—two in the U.K. and one in India—hope to put that view to a stringent test within the next year or two with an intriguing experiment that ultimately aims to determine whether or not large objects obey the strange rules of quantum theory.
The experiment, described in a recent issue of Physical Review Letters, harks back to a conundrum vividly framed nearly a century ago by Erwin Schrödinger, one of the founders of quantum mechanics. What would happen, Schrödinger asked, to a cat trapped in a closed box with a vial of poison that has a 50–50 chance of shattering and killing the cat? According to quantum mechanics the cat is at once alive and dead, existing in both states until someone opens the box and looks inside. That’s because it’s only when an observer makes a measurement of the system—opens the box and checks—that the two possibilities have to collapse into one, according to quantum theory. The story is meant to illustrate how applying these quantum rules to big things—basically, anything visible to the naked eye—leads to absurdities.
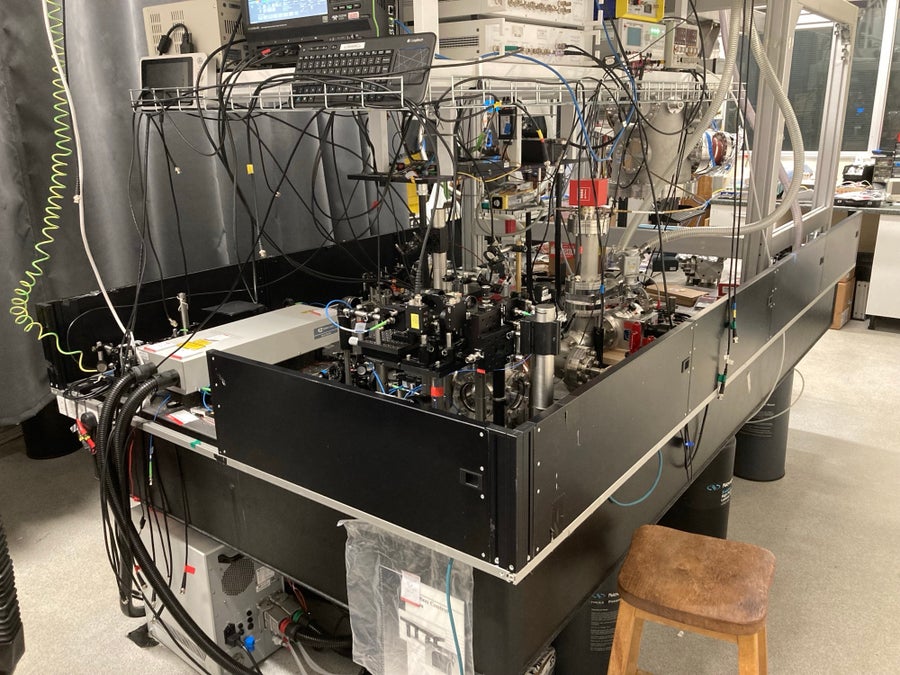
An experimental setup with optical levitation in Hendrik Ulbricht’s research lab at the University of Southampton in England.
Credit: Tiberius Georgescu
So if quantum mechanics is true—and it has been a phenomenally successful theory for predicting the behavior of particles—why do we never see cats that are both dead and alive? Do the laws of quantum mechanics break down at a certain level? Some physicists see that as a possibility. But most would argue that the apparent absence of quantum effects in our own experience of the world arises because the countless interactions of atoms with the surrounding environment blur the true nature of things. As a result we perceive a kind of dumbed-down, nonquantum version of reality.
If that’s the case, then a carefully designed experiment that isolates an object from nearly everything in its environment should allow physicists to glimpse the actual quantum behavior of that object, even if it’s relatively large. That’s the goal of the experiment proposed by Bose, Debarshi Das, also of UCL, Hendrik Ulbricht of the University of Southampton in England and Dipankar Home of the Bose Institute in India. “There are two possible outcomes,” Home says. “One is that quantum mechanics is valid [at all scales. The other is that] there is a region where quantum mechanics does not hold.”
Most of the hardware needed for the experiment is already in place and fits on a tabletop in Ulbricht’s lab. (He’s the lone experimentalist of the group; Home, Das and Bose are theorists.) The experiment will use lasers to suspend a single nanocrystal of silica—a microscopic glass bead—as it oscillates around the focal point of a small parabolic mirror carved out of a block of aluminum housed in a vacuum chamber. Although the bead is only about 100 nanometers in diameter—roughly the size of a virus—it is nonetheless at least 1,000 times larger than the clumps of molecules that until now have set the experimental benchmark for “quantumness.”
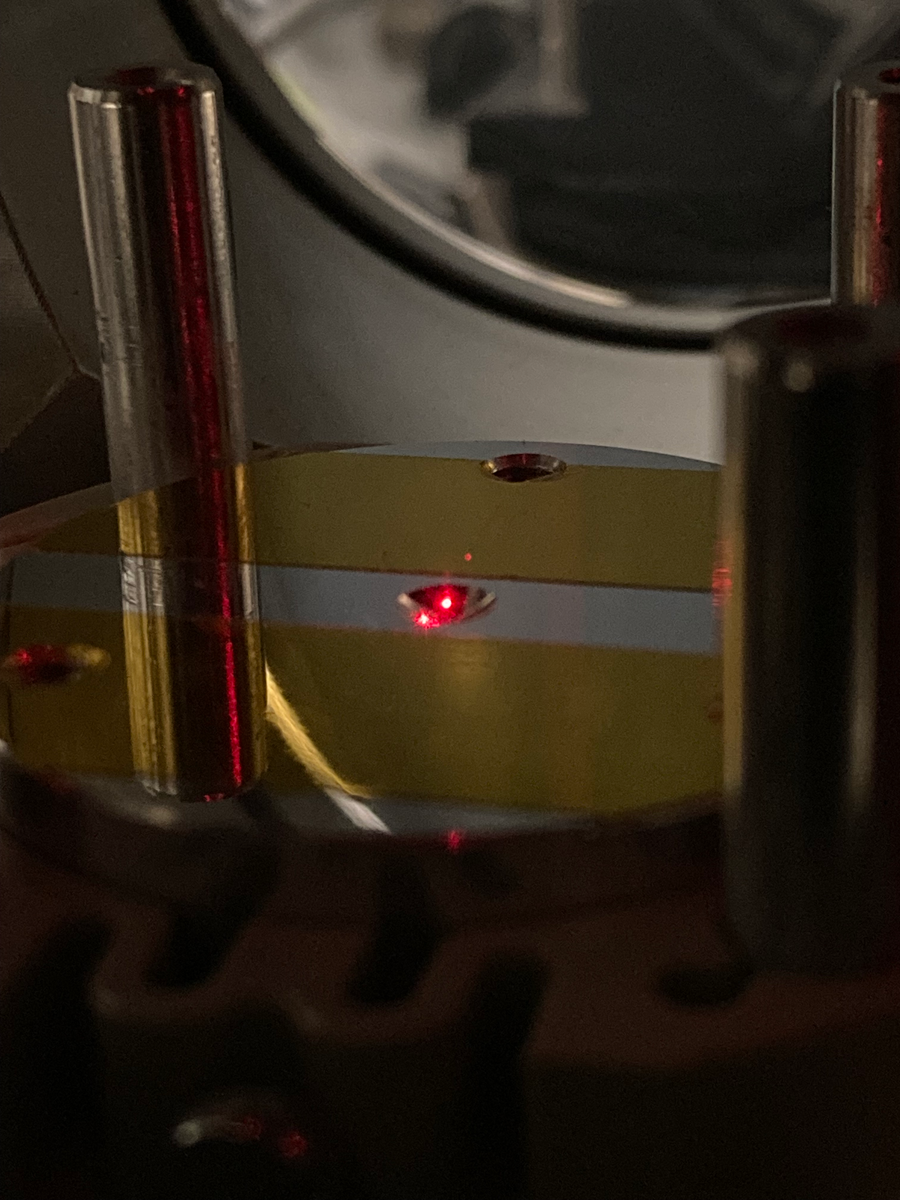
An illuminated red silica nanoparticle in an optical parabola trap at Ulbricht’s lab.
Credit: Tiberius Georgescu
For all its technical complexity, the experiment mimics a very simple phenomenon: the motion of a pendulum. An electromagnetic field drives the silica bead back and forth. Like a metronome, the bead regularly ticks from point A to point B and back again. As far as classical, nonquantum physics goes, that should be the end of the story. But a quantum pendulum should behave very differently. Its position will change depending on whether or not someone is watching: it might start at A but end up somewhere to the left or right of B. Call it Schrödinger’s pendulum.
The experiment will test the very nature of reality: Is it completely objective, or do our own observations play a role in creating what we see? To find out, the experiment will be run in two slightly different ways. In one version, a laser will be aimed at a spot where classical physics predicts the bead to be, say, at position B. If the bead is indeed there, it will reflect the laser light back to a detector. In the second case, the laser will be shined twice: first at an intermediate position and then a second time a little later in the bead’s path. According to classical physics, the intermediate measurement should not affect the subsequent position of the bead—it should always end up at B. After all, in daily life we can’t change the movement of a metronome simply by looking at it.
But in the quantum case, that intermediate measurement has a profound effect. As with Schrödinger’s cat, the bead doesn’t actually exist in any fixed state until it’s observed. Before that, the bead can’t be said to be anywhere at all; it’s just a cloud of possibilities and assumes a definite position only when measured. The mere act of observing the bead at one moment in time changes where it will be at a later moment when the laser shines the second time. If the rules of quantum mechanics hold, the bead may sometimes be found at B, but sometimes it won’t be.
“When you measure, you create that reality,” Bose says. “In quantum mechanics the thing does not exist in a particular place before that. There is no truth before you measure.”
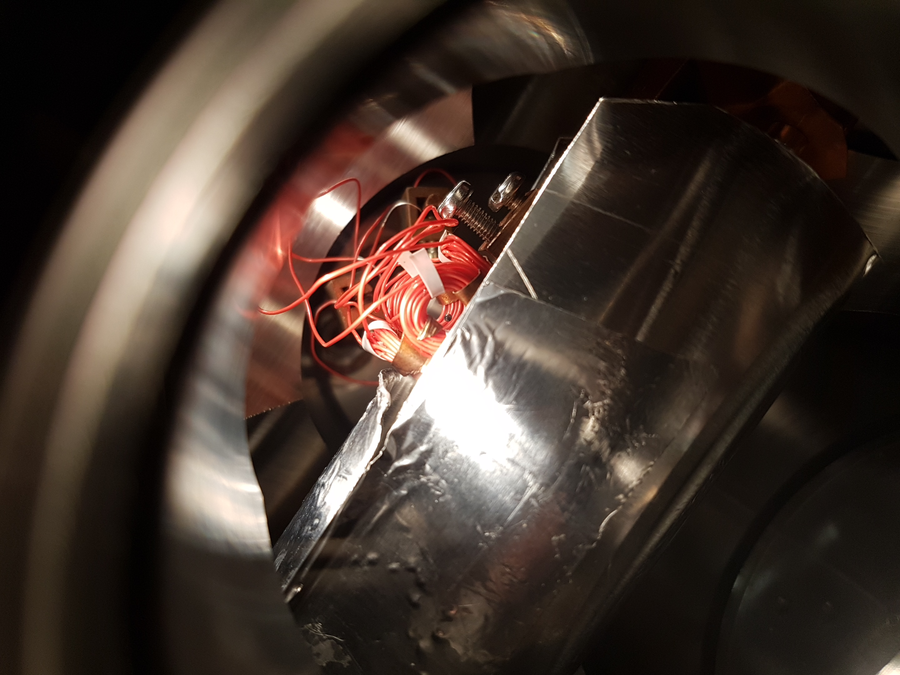
Particle in magnetic trap with field coils in Ulbricht’s lab.
Credit: Marion Cromb
For statistically robust results, Ulbricht will have to work quickly and make about 100,000 measurements of the bead over the course of an hour. (The longer the experiment runs, the greater the risk that slight temperature changes or other subtle effects might interfere with the quantum aspects of the setup.) At the same time, he’ll have to calibrate the position of his detectors so that they count only photons that interact with the bead and not any that might bounce off the small parabolic mirror in the aluminum block.
In principle, Bose, Ulbricht, Das and Home believe that their experimental approach could eventually be scaled up to work with much larger objects, perhaps even with something as massive as a few kilograms. But in that case the array of potential contaminating effects, or “noise,” would become far more difficult to control. “Noise scales very badly [with size],” says Vlatko Vedral, an experimental physicist at the University of Oxford, who also studies the classical-quantum divide. “It scales exponentially. Would I be surprised if this could be done? I don’t know—it’s not a trivial experiment.”
If the current experiment turns out to violate the predictions of classical physics, it will bring the quantum world almost palpably close to our own. “We believe that quantum mechanics is a universal theory,” Das says. “The theory itself does not have any limit. But in reality, whether that is true or not, we don’t know yet. Only experiment can resolve this dilemma.”